Gene therapies, how to deliver genes
Gene therapies represent groundbreaking therapeutic approaches that introduces genetic material into cells. The therapies enable to restore lost or damaged functions, strengthen biological systems, or correct defective genes. Because genes cannot easily enter cells on their own, delivery systems are essential for transporting them. Among these, virus – natural specialists in gene delivery – is the most promising one.
Adeno-associated viruses (AAVs) are the gold standard for clinical applications due to their efficiency. However, these viral delivery systems face challenges such as immune reactions, safety concerns, and high treatment costs. To address these issues, researchers are exploring alternatives, including endogenous virus-like systems embedded in our genome or non-viral delivery methods.
Non-viral approaches use chemical or physical mechanisms to deliver genes, employing substances such as lipids, polymers, peptides, inorganic materials or stimulation such as electronic pulses, plasma, or ultrasound. While these systems offer potential advantages in safety and cost, their lower delivery efficiency compared to viral systems remains a significant barrier. Overcoming this limitation is critical for advancing non-viral approaches into clinical applications.
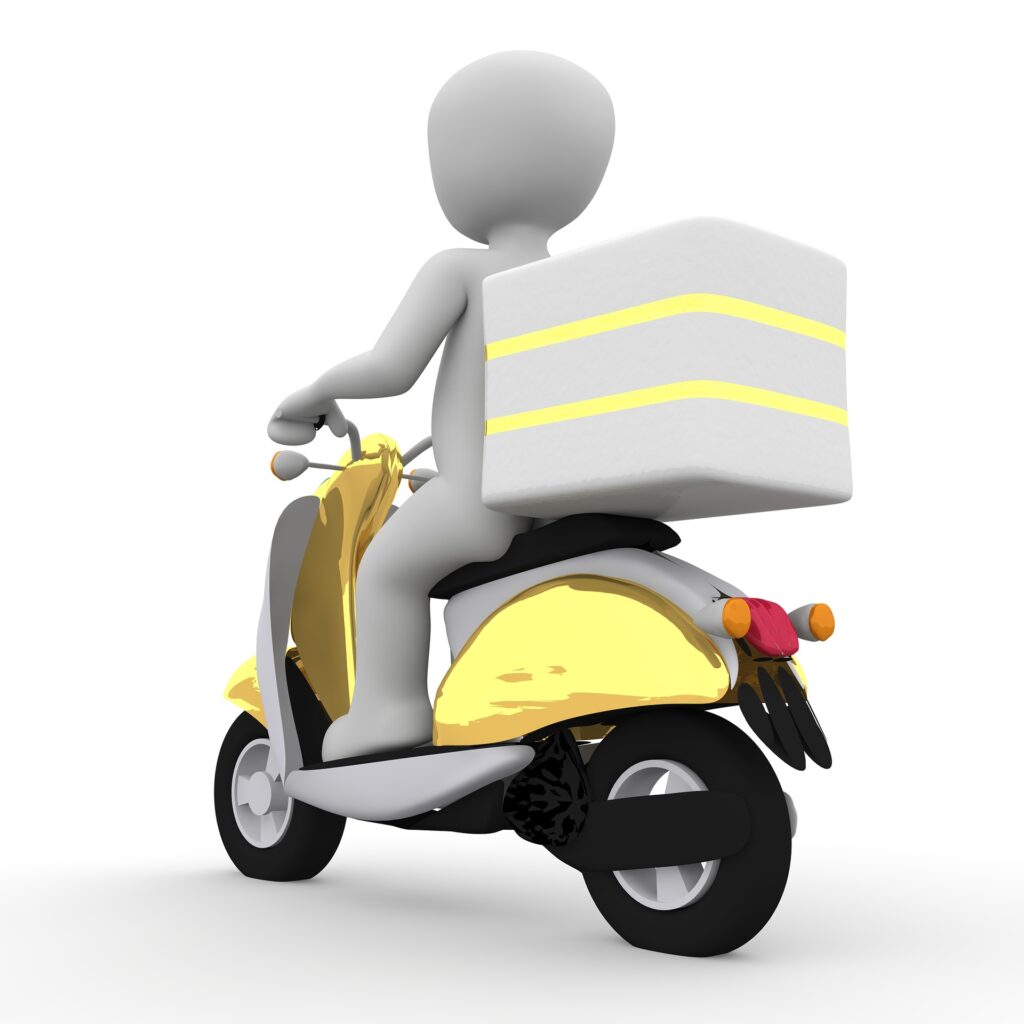
Gene delivery vectors
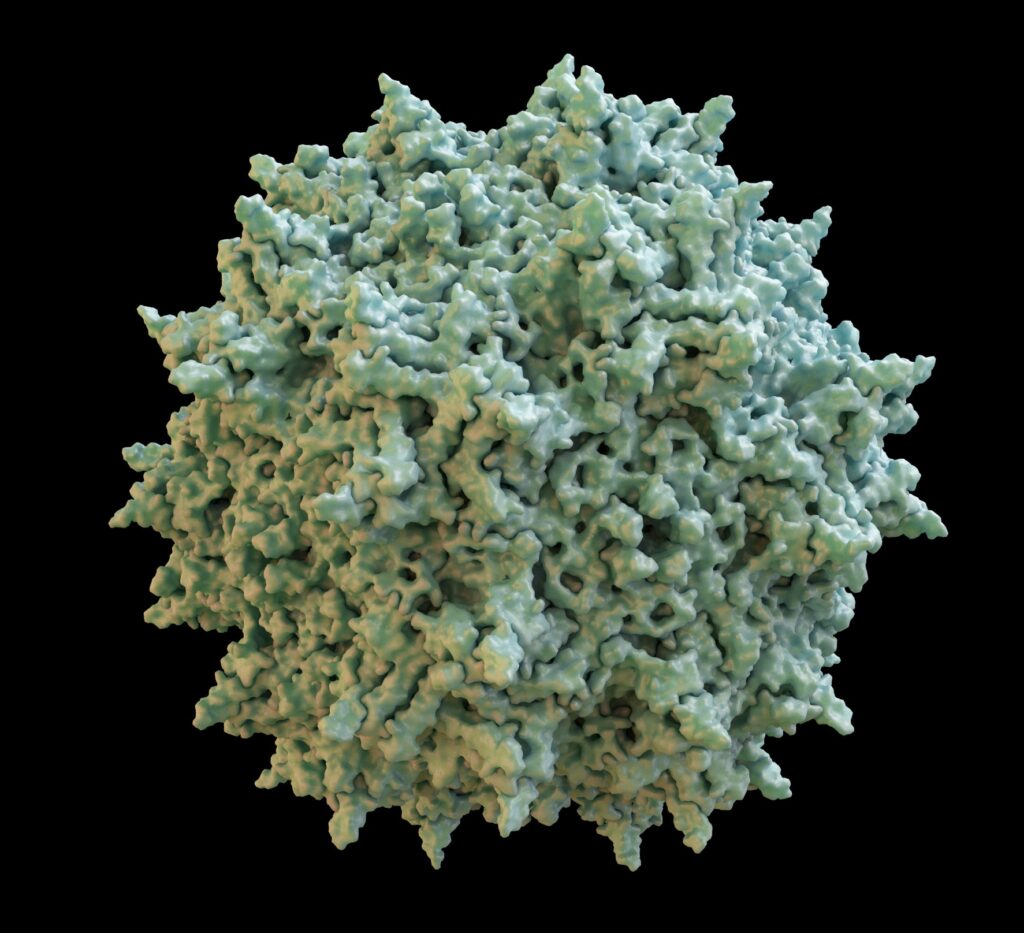
Gene delivery vectors are systems designed to transport genetic material into cells. They typically consist of the gene of interest, expression control elements (such as promoters and regulatory sequences), and a delivery carrier. Plasmids, which lack dedicated carriers, are exceptions and are still classified as vectors. There are two main categories of vectors: viral and non-viral. Viral vectors commonly used in gene therapy include retroviruses, lentiviruses, adenoviruses, adeno-associated viruses (AAVs), and herpes simplex viruses (HSV). In contrast, non-viral vectors generally rely on nanoparticles composed of lipids, peptides, polymers, inorganic materials or DNA nanostructures as alternatives to viral capsids. Additionally, novel add-on technologies have been developed to enhance the functionality of gene delivery systems. These include mechanisms to regulate gene expression, such as RNA-based on/off switches, mRNA degradation codes, and synthetic cis-regulatory elements.
Plasmids and other related DNA vectors
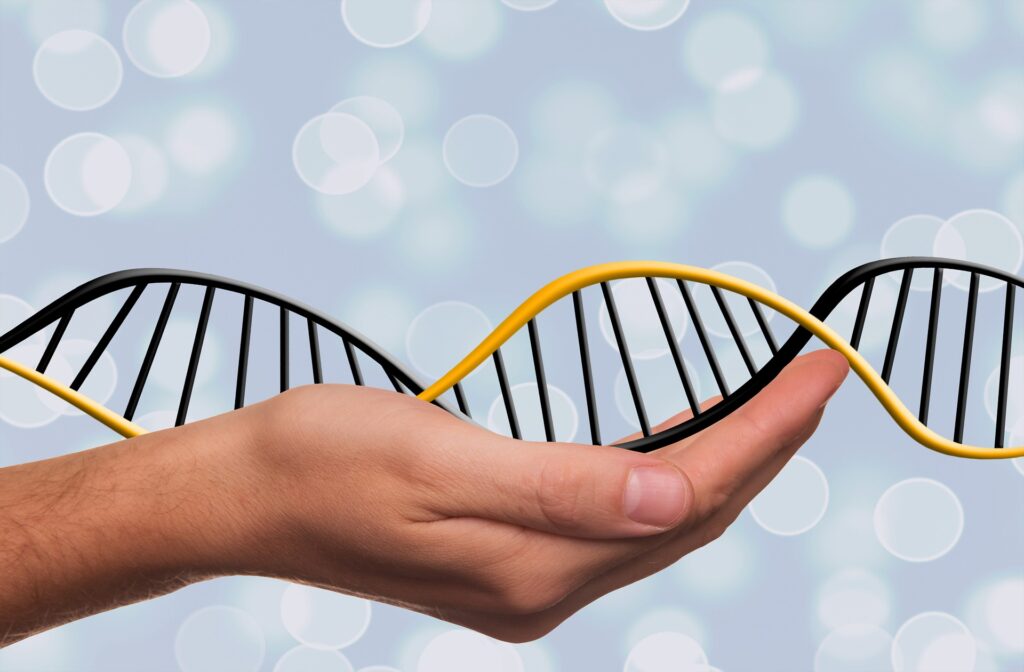
Plasmids—originally bacterial extrachromosomal double-stranded circular DNA—have traditionally been widely used as DNA vectors. Conventional plasmids typically include antibiotic resistance (AR) genes in their backbone as selection markers for production purposes. However, regulatory agencies discourage the use of AR gene-containing vectors for human applications. In response, novel plasmids and related vectors without AR markers have been developed, such as minicircles, NanoplasmidTM, miniVec™, and dbDNA. These advanced vectors often demonstrate superior gene expression profiles compared to conventional plasmids, likely due to the absence of bacterial genes, which can trigger transgene silencing. Gene expression from these vectors is generally transient, lasting for a short duration in replicating cells and a medium duration in non-replicating cells, as they remain episomal (separate from chromosomal DNA) and do not integrate into the genome. As a result, these non-viral DNA vectors are considered a safer alternative for therapeutic applications.
Gene electrotransfer
Gene electrotransfer (GET) is a promising physical method for gene delivery, significantly enhancing the efficiency of gene transfer into cells. It has become widely used, particularly for in vitro applications such as the manufacturing of cell therapy products. In vivo, GET has been explored in numerous clinical trials, including those focused on vaccines for infectious diseases (such as COVID-19) and cancer immunotherapy. While gene transfer via electroporation was initially believed to rely on cell membrane permeabilization through pore formation, accumulating evidence suggests that endocytosis may play a significant role in DNA entry into cells. Despite its potential, GET faces challenges, particularly in in vivo applications, where transfection efficiency remains a limiting factor. Overcoming this obstacle is crucial for translating GET from basic research into clinically viable treatments.
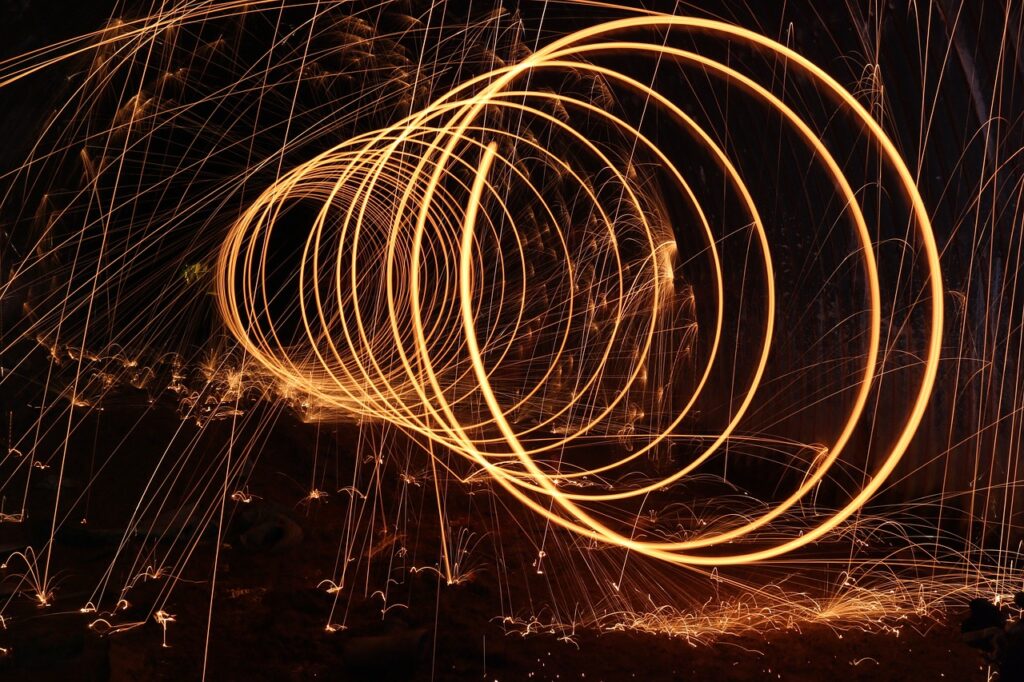
Approved gene therapy products
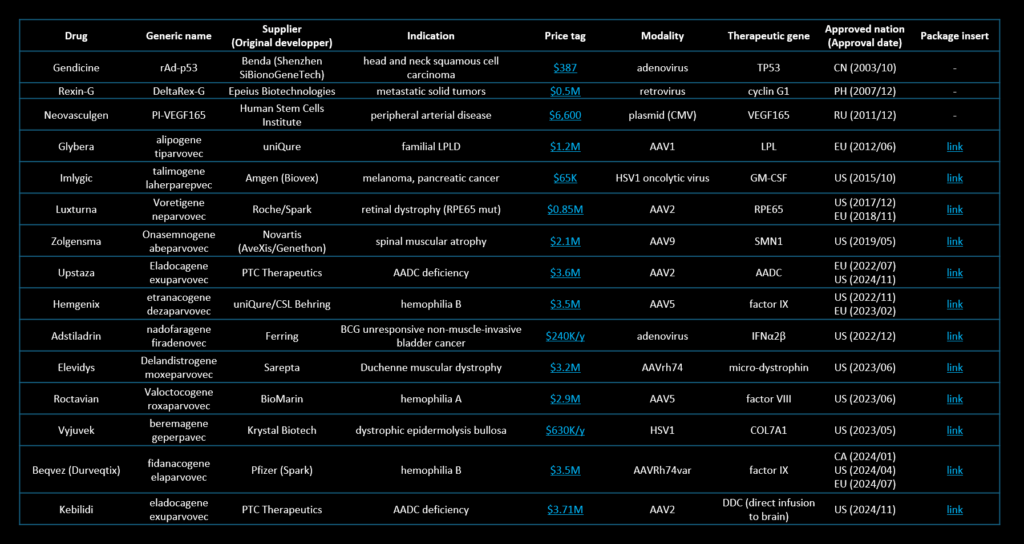
The table on the right lists approved in vivo gene therapy products as of December 16, 2024, excluding genetically-engineered cell-based products. Notably, the number of approved in vivo gene therapy products has increased significantly in recent years, reflecting the rapid expansion of the gene therapy market. Please find the PDF version of the table with an active link at the link below.